CFS conference bridges physics gaps for a better SPARC tokamak
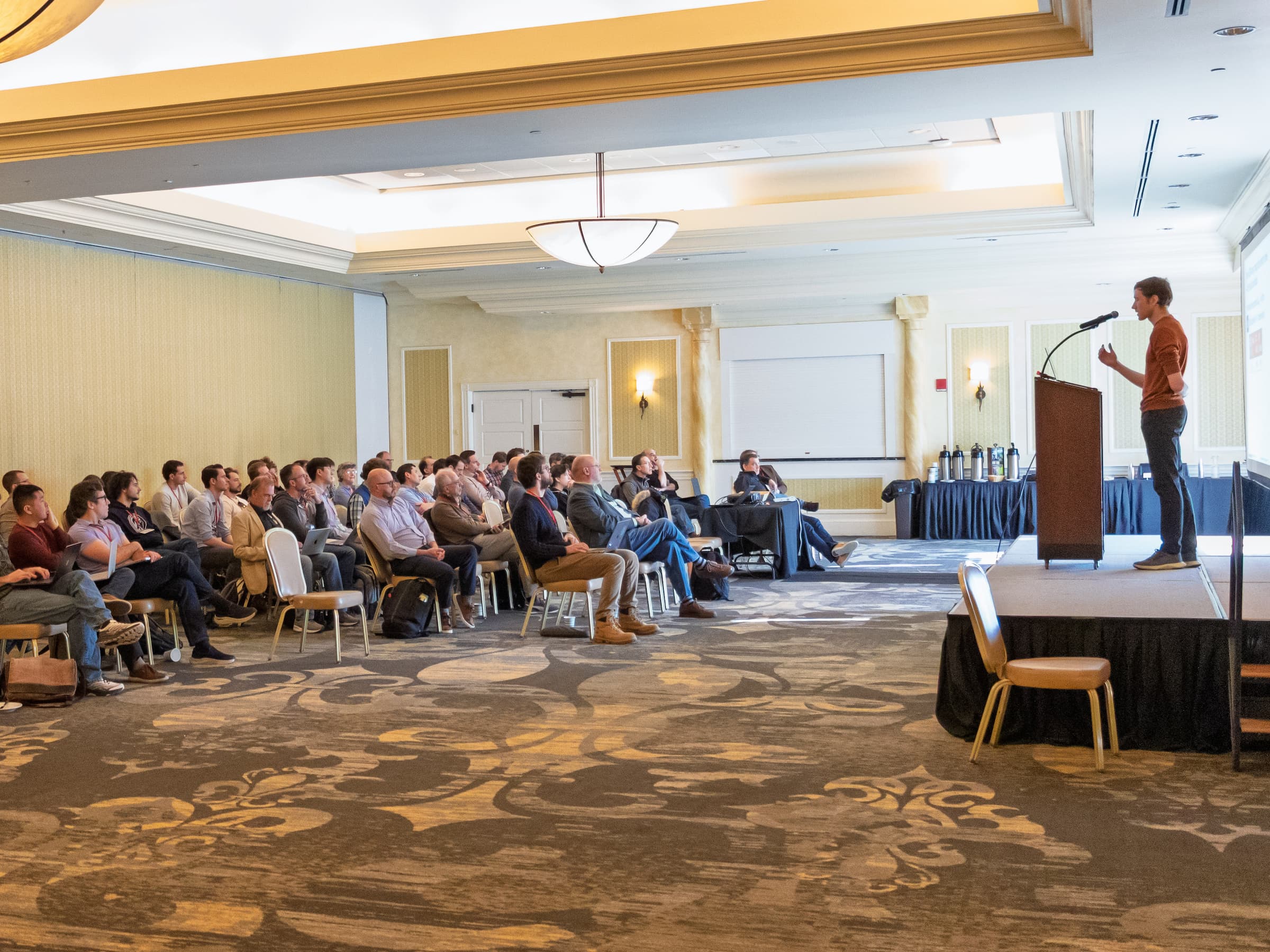
To operate our first fusion energy machine, SPARC, we’ll have to perform a balancing act. The heart of the machine must reach extremely high temperatures to drive the fusion process. But not far away from that, there’s a much cooler region — the boundary with the more ordinary world of metals and magnets.
To connect these domains, we need a collection of experts who understand the tradeoffs and constraints. That’s why Commonwealth Fusion Systems (CFS) recently gathered about 60 experts in a meeting called the Boundary Collaborators Workshop — the first time CFS has organized such a sizable event.
For a week in March, plasma physicists joined experts in related areas like machine control and diagnostics at our headquarters in Devens, Massachusetts to hear of each others’ research and learn how it applies to SPARC.
“Getting these people together in one big room offers the possibility for people to connect,” said Thomas Eich, a CFS physicist who leads the SPARC divertor and boundary physics team. And in his estimation, the workshop succeeded in building those connections.
The workshop is an example of CFS’ open innovation approach, partnering with leading experts from around the world to tackle the tough parts of commercializing fusion energy. These collaborations have helped to solve challenges not just for SPARC, but also for the broader fusion community.
The workshop was crucial for setting the next steps of physics collaboration on SPARC and its power plant successor, ARC. After hearing CFS strategies for boundary controls and power exhaust, workshop attendees discussed remaining challenges and began developing a research roadmap to address them.
Fusion energy, released when lighter atoms like hydrogen combine into heavier ones like helium, works at temperatures hotter than the center of the sun. That’s what we’re aiming to do with our SPARC fusion machine, which is designed to heat fusion fuel past 100 million degrees Celsius (180 million degrees Fahrenheit) into a highly energetic cloud of charged particles called a plasma.
Creating the superhot plasma core is only one challenge. What happens at the edge of the plasma is also critically important. Here, plasma meets the solid walls of SPARC. Our boundary collaborators focus on this interface layer — finding ways to keep the plasma as hot as possible while keeping the walls cool.
SPARC is a tokamak, a donut-shaped fusion device that’ll use extraordinarily powerful magnetic fields to confine and control fusion fuel that’s heated into a plasma. By heating the core of the plasma to extremely high temperatures, SPARC will make its hydrogen ions energetic enough to fuse into helium when they collide. The tokamak’s magnetic field will confine the ions and electrons that make up the plasma so they stay put. The magnetic field acts like extremely effective insulation for the hot plasma, letting the plasma stay hot and not touch the machine’s hardware.
This insulation isn’t perfect, however, and some of the plasma leaks out because of processes like turbulence. At some point, the plasma reaches the solid walls of the device in a component called the divertor — a zone specifically designed to handle the heat and particles coming out of the plasma.
“The divertor is like the trash can,” quipped Rebecca Masline, a postdoctoral fellow who studies the subject at the Massachusetts Institute of Technology and who attended the workshop. “It diverts the exhaust that comes off the core into a special region where we can handle it.” The divertor also cleans out helium “ash” from fusion along with other impurities.
That might not sound glamorous, but it’s essential. Without the divertor handling the heat coming out of the plasma, we wouldn’t be able to reach and sustain the temperatures we need for fusion.
To deal with the hot plasma, SPARC’s divertors — one toward the top of the plasma-containing vacuum vessel and another toward the bottom — will be lined with tungsten, a metal that can handle extreme temperatures. Special magnets in SPARC also will help steer the plasma exhaust to direct its heat where we want it within the divertor.
The divertor and core are often at odds. SPARC’s core needs to be as hot as possible to maximize the rate of fusion, while the divertor needs to stay as cold as possible. The workshop’s job was to help reconcile these two realms.
“We have to close this gap,” Eich said.
For example, neon and argon impurities in the plasma help radiate heat, making the divertor’s job easier. But adding too much of them cools down the plasma core as well, undermining the fusion process in the tokamak.
SPARC’s divertor has a particular challenge. Our magnets use high-temperature superconductors (HTS) whose stronger magnetic fields enable higher performance in a smaller tokamak. That’s good for making the machine more compact and affordable, but it also means that the heat is concentrated more intensely on the divertor.
MIT’s Masline said she found the workshop useful — for example hearing about details of SPARC diagnostics that will help her design her computer models, and meeting others at MIT’s Plasma Science and Fusion Center and from around the world. She presented two talks at the workshop, both involving computer models of complicated physics like the effect of charged particle drifts in SPARC’s divertor.
“I spend a lot of time on my computer doing my simulations,” Masline said. “It was useful to get to connect with all those people I don’t always speak to directly — people from across the world or sometimes just across the hallway.”